An advanced perovskite-silicon solar cell has been tested in various weather conditions. The tests have clarified how the current-voltage characteristics of tandem roof-mounted panels will differ from the corresponding parameters obtained in the laboratory.
The design of innovative solar cells is usually optimized for laboratories. The panels are tested and improved under ideal conditions in which lighting and temperature are stable.
But in the real world, things are different. The angle of incidence of the sun’s rays constantly changes during the day, and clouds continually reduce the intensity of the incoming light. Therefore, the heating of the solar cells inside the panel varies as a function of two variables – the height of the Sun and the weather. And because of the varying temperature of the panel, its performance – the output of energy – in the field will not be the same as in the laboratory.
Mathematical models have been developed for conventional solar cells to show what these differences will be. That is, knowing the efficiency of a conventional silicon solar panel calculated in the laboratory, it is possible to predict with high accuracy how much electricity it will produce when installed somewhere on the roof of a house in the Odessa region.
However, how revolutionary solar modules , such as perovskite -based tandem panels, will perform under natural conditions has remained a mystery until recently. It was not clear exactly how changes in the solar spectrum and, more importantly, temperature fluctuations would affect their performance.
A recent scientific publication in the journal Nature Energy by Erkan Aydin, Stefaan De Wolf and their colleagues from Saudi Arabia and Spain helped shed light on these ambiguities. The researchers determined how relationships between temperature and band gap energies affect the performance of 2-terminal perovskite-silicon tandem solar cells under real-world operating conditions. In particular, they found that the tandem panel performs most efficiently at lower temperatures than previously thought .
Reference: in tandem perovskite-silicon cells, a layer of perovskite is deposited on top of traditional silicon. The two working substances allow the panels to collect more solar energy. A translucent perovskite photocell captures certain wavelengths from the visible spectrum and passes other wavelengths through itself for disposal by the silicon cell.
A two-terminal tandem cell works best when both of its photocells have the highest equal current density. If the cell perovskite and silicon layers work with different efficiency, then the performance of their tandem is reduced to about the level of the weakest of its two links.
The current density of a photocell is a value that depends on the band gap of the absorber (silicon or perovskite). And this parameter is unstable in the field. The bandgap width can increase or decrease as weather conditions change.
By investigating the spectral sensitivity of a tandem cell by measuring its external quantum efficiency, Erkan Aydin’s team found that the band gap of a perovskite photocell increases with heating (Fig. 1a). This means that the current density generated by the cell’s perovskite photocell decreases as it heats up.
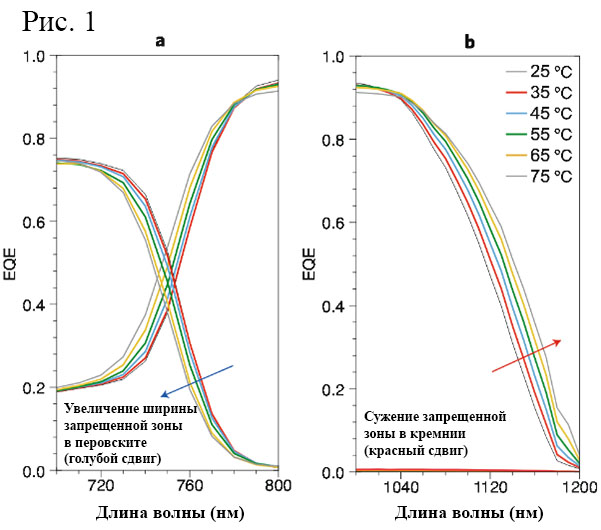
With regard to the silicon photocell of the cell, the researchers described two effects that increase the current density in it. First, increasing the perovskite band gap allows more photons to pass to the silicon cell. Second, increasing the cell temperature narrows the silicon band gap (Fig. 1b). Due to these effects, the two photocells of the experimental tandem cell, which demonstrated the same current density in the laboratory, worked differently in the open air (Fig. 2).
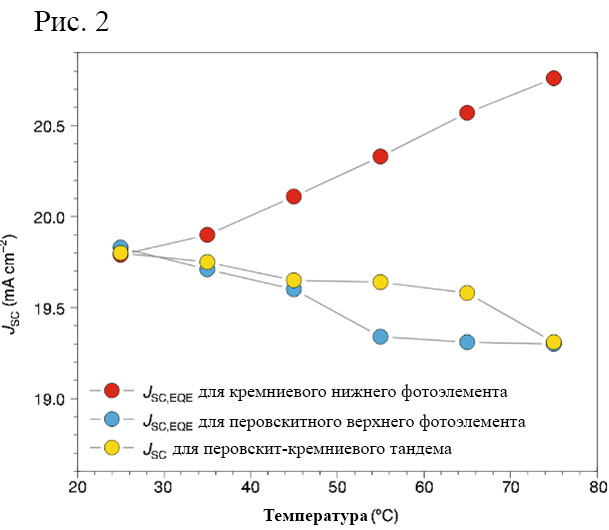
The researchers found how the band gap energies of perovskite and silicon depend on ambient temperatures. To do this, they calculated the parameters of their tandem cell, first in the laboratory, and then measured its current-voltage characteristics and temperature outdoors in a hot and sunny climate. There, their experimental device heated up to 60°C from the Sun, but, according to the researchers, the temperature inside the tandem cell could rise to 75°C if solar panels were placed on rooftops.
Erkan Aydin’s team confirmed the results of previous studies showing that an increase in temperature leads to a decrease in voltage. The researchers also calculated that the optimal perovskite band gap of 1.73 eV, which was theoretically determined under ideal conditions, is too high. Under the temperature conditions under which they conducted their field experiments, the best energy yield was obtained when the band gap was 1.685 eV. But Erkan Aydin’s team hypothesizes that future research could shift the ideal value of this parameter even lower, closer to 1.65 eV.
The fact that the optimal energy yield-maximizing band gap turned out to be smaller than the theoretical one is good news. Formerly “high-bandgap” perovskites (with bandgap> 1.7 eV) were associated with device stability issues. Now there is more confidence in the imminent appearance of reliable and durable perovskite-silicon solar panels.
Erkan Aydin’s team has improved understanding of how outdoor temperature changes affect perovskite and silicon band gaps and affect tandem device performance. However, the full picture of how the perovskite-silicon cell performs in the field needs to be unlocked to make this technology commercially and technically feasible.
More research is needed to elucidate how the parasitic absorption of the perovskite layer reduces its optimal band gap. You also need to test perovskite with a textured surface in weather conditions. It may prove to be a more suitable material for making solar cells. And its optimal band gap will also not be the same as that of perovskite with a smooth surface.
By studying these factors and their interactions with temperature and meteorological conditions, researchers will be able to accurately model the “field” performance of a perovskite-silicon cell. This model is important for understanding how a tandem cell should be designed to best output energy in real-life solar panel conditions.